Since 2016 a volunteer position I’ve held is to chair the exhibits committee of the International Spaceflight Museum, which started out in Second Life in 2006. It’s mission is to build and exhibit full scale models of all the world’s rockets and spacecraft. It grew to encompass two simulator regions in the SciLands estates in Second Life. It incorporated as a Texas 501c3 nonprofit with a mission to educate the public and particularly school children about the history and future of space exploration, starting with the original pioneers like Robert Goddard, Yuri Gagarin, Neil Armstrong, and their rockets, to the present.
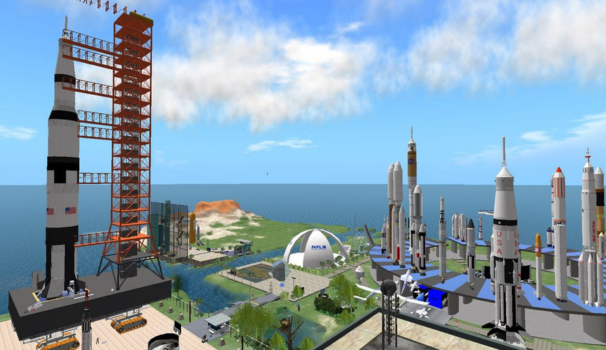
The International Spaceflight Museum has since expanded into OpenSim, an open sourced version of Second Life, which you can access via the same viewers — though not the official one from Linden Lab — that you use to access Second Life, including the most popular viewer, Firestorm.
OpenSim has over 600 independent grids, most of which are connected to the hypergrid — a connectivity tool that allows users to teleport their avatars from one grid to another, with the same appearance, inventory, and identity. Some of these grids rival Second Life in land area, like OSgrid, the oldest and most popular, and Kitely, a commercial grid that provides cutting edge service and stability as well as active community involvement with management.
The International Spaceflight Museum’s facilities in OpenSim are in Kitely, where it has the a land area larger than 64 Second Life regions, for a tiny fraction of the cost. This has allowed us to expand far beyond the limited space we can afford in Second Life. The hypergrid address is grid.kitely.com:8002:ISMuseum.
Our main museum space is equal to four Second Life-sized regions. We also have a separate sandbox. In addition, we have been building a Mars Base that is more than a half kilometer in diameter using the latest mesh physically-based rendering designs created largely by myself. The goal is to provide a space for those dreaming of migrating to Mars a taste of what to expect and an opportunity to train virtually for the experience, as we are building in functionality into all equipment on the base so that virtual analog astronauts may train to gain expertise on all aspects of base construction, maintenance and management.
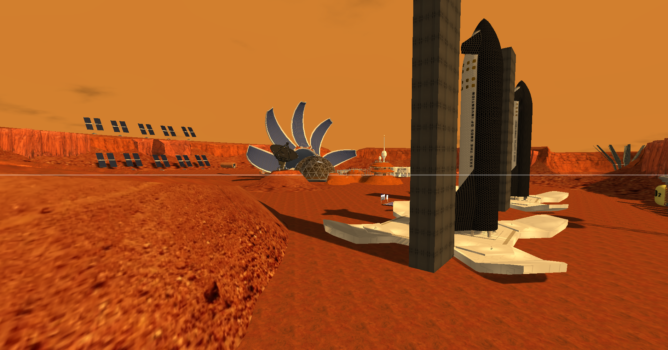
This includes training on building-sized 3D printers, tunnel boring machines, regolith mining and refinery equipment, high intensity agriculture and mariculture, maintenance of Hyperloop trains, solar, wind and nuclear power systems, as well as full scale SpaceX Starship rockets and the Sabatier Process in situ resource utilization fuel production systems that will provide them the ability to return to Earth to pick up more colonists.
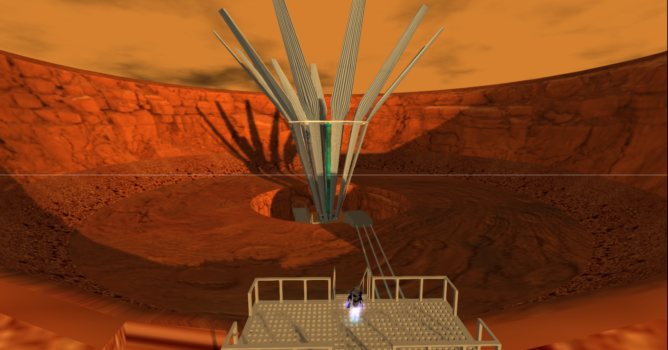
In situ resource utilization is a broad range of essential technologies that allows colonists to build almost all of the facilities and equipment they need to colonize from native resources found on Mars. That includes the ferrous minerals used to produce steel, silicates to produce glass, carbonates to produce marscrete, graphene from atmospheric CO2 for superconductors, and the rocket fueling the Starship rockets.
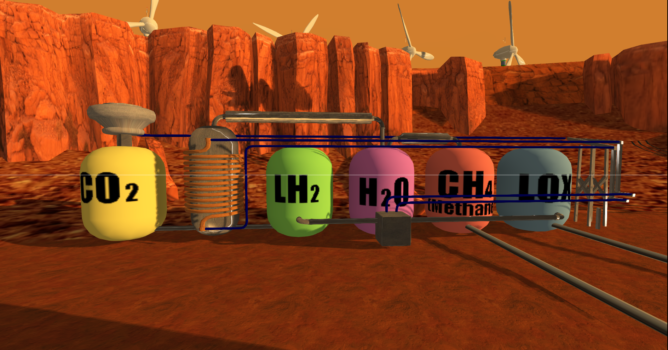
The Sabatier process is a chemical engineering process first developed in the 19th century. It can be used to compress atmospheric CO2 via a turbo compressor, located over the CO2 tank in the image above. Water found on Mars in abundance as ice can be electrolyzed into liquid oxygen and liquid hydrogen. Then the liquid hydrogen is combined with the CO2 in the reactor chamber seen between their two tanks. This chamber has catalytic elements in it made from platinum coated material, and is surrounded by a powerful induction coil to heat the combined solution to heat it to high temperatures so the combination catalyzes into a mixture of gaseous methane and steam. The mixture is cooled in a condenser to separate the water out first, with the remaining gas going through a further condensation step to become liquid methane.
With these in situ resource utilization materials the elements of the colony can be built. That includes the steel needed to produce the frames of the modules, marscrete infused with graphene to strengthen the mixture to over five times that of earth concrete to encase the metal frames, steel piping, graphene cables, and so on. Water from Martian ice is suppled to agricultural modules that grow food to sustain the population.
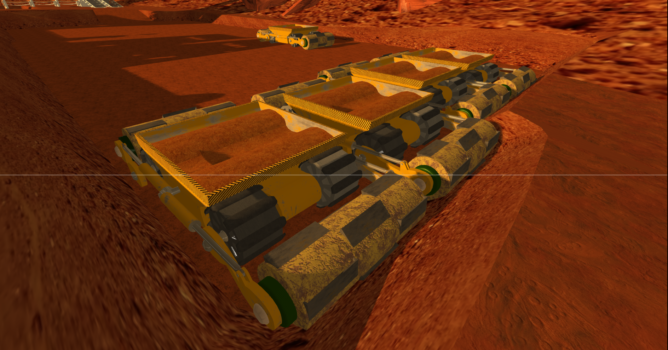
RASSORs — which stands for Regolith Advanced Surface Systems Operations Robots — carry out the in situ resource utilization materials process to build the base, scooping up Martian regolith in the drums fore and aft of the cart. When the drums fill, they raise up over the bins and reverse direction to empty their contents into the bins. When the bins are full, each RASSOR returns to the regolith refinery to deliver its payload.
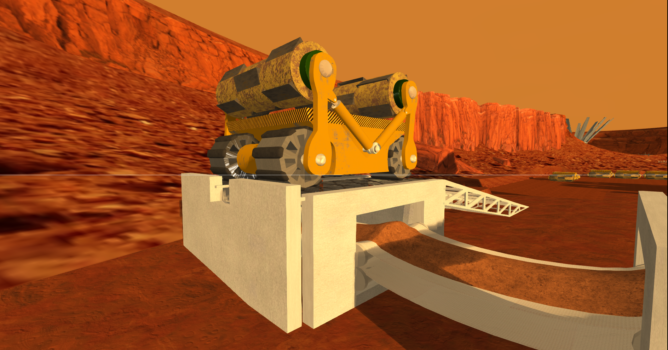
The first modules will be built on the Martian surface, then covered with ample amounts of regolith to provide greater radiation shielding.
Radiation levels on Mars surface are slightly higher than experienced at the International Space Station. Mars is 40 percent further from the Sun so it receives less solar radiation overall, but it is not protected by a magnetic field like Earth is. As a result, Earth’s surface receives 40 percent less sunlight than is received in low Earth orbit, but most of what is filtered out are harmful UV rays, solar cosmic rays, and also galactic cosmic rays.
The unshielded habitation on Mars surface is utlimately sterilizing and deadly, but nobody is working on Mars in shirtsleeves.
A foot of regolith covering pressure modules is quite sufficient to lower radiation levels to below that which we get on Earth. Leaded glass can also block out most harmful radiation, as seen on this dome in the mage below, that is used for high intensity agriculture. The shell behind the reflects more sunlight into the dome to boost photosynthesis, yet it is backed by regolith foamcrete to provide radiation protection from the rear, while the large solar panel petals can fold down to enclose the dome and provide increased protection.
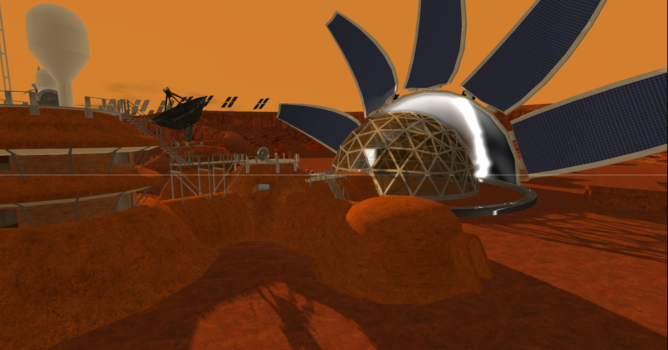
The Universal Habitat Dome shown above is used for high intensity sustainable agriculture. A 40-meter diameter facility can grow enough food to feed between 12 and 20 people, with plant and animal waste being recycled into the system to produce rich fertilized soil.
Once tunnel boring machinery is assembled on Mars, colonists can begin burrowing under the surface for even greater radiation protection over the long term. As tunnel boring machines bore 6-meter- diameter tunnels, 3D printing machines will follow behind, assembling the pressurized tunnel modules to fit within the bare tunnels. Modules to provide additional sleeping, storage, living, growing and working spaces for a burgeoning population.
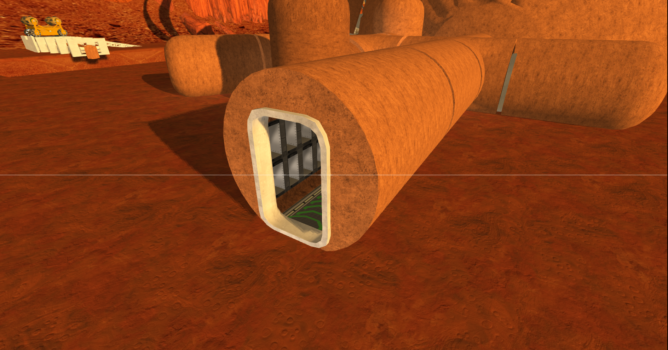
Once multiple colonies are constructed on Mars, travel between them cannot be done across the surface, or via air travel. Thus, the tunnel borers will dig Hyperloop tunnels over thousands of miles to connect these disparate colonies so that they may trade amongst each other, allow for migration of populations to new colonies, and ultimately, commuting from outlier settlements into larger urban communities.
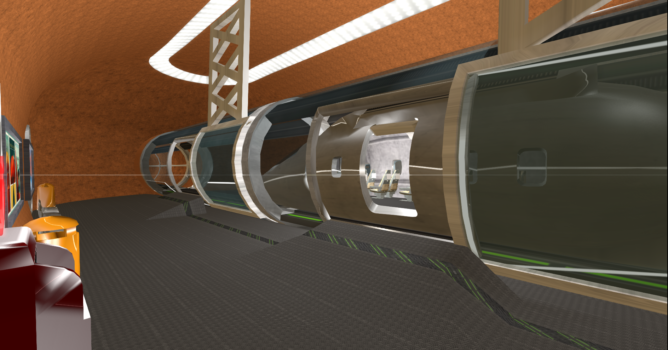
The Hyperloop train enables mass transit between communities for passengers and cargo. Powered by electricity generated from solar, nuclear, and wind sources since there is no oil on Mars, powerful superconducting magnets using graphene conductors for cheap room temperature superconduction levitate the Hyperloop train and accelerate it to over 600 miles per hour inside the tunnel tubes, which will be maintained at a vacuum to limit atmospheric drag. The tubes within stations will be closed off via airlock doors.
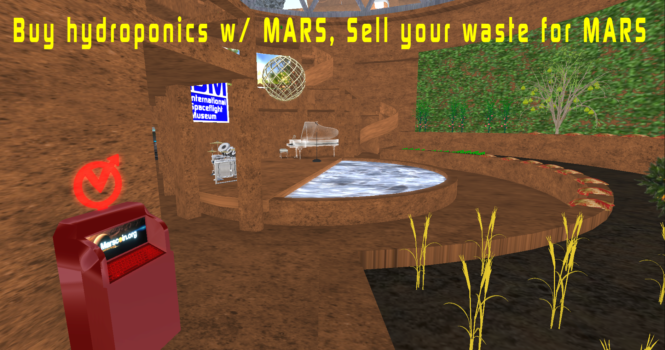
Terraforming Mars
All of this effort is entirely doable, with current day technologies. The real question is, can we make Mars habitable on its surface like Earth is? The short answer is yes.
The first step goes back to the in situ resource utilization technologies. There are ample chlorine and fluorine based minerals on Mars’ surface. Along with atmospheric CO2, these can be catalyzed by basic chemical engineering into chlorofluorocarbon and carbon tetrafluoride, both of which are extremely strong greenhouse gasses.
I have previously calculated that if we built facilities to produce chlorofluorocarbons at the same rate they were produced on Earth prior to the Montreal protocol, and maintained this production rate, dumping the chlorofluorocarbons into the Martian atmosphere, within 30 years enough warming would take place to cause a runaway outgassing of CO2 from ice and regolith deposits deep underground.
As more CO2 outgasses, the warming continues. Within another 30 years, Mars atmospheric pressure will rise to somewhere between 300 and 500 millibars, which is about the same pressure we see in Tibet. So we won’t need spacesuits anymore, though we will need oxygen masks, to walk around outside in shirt sleeves. Climate will be temperate or warmer across 75 percent of the planet, and the northern third of the planet will be covered in a sea with an average depth of more than half a mile.
With the ice melted to water, and open seas on Mars, then the hydrological cycle can begin. Water will evaporate under temperate and tropical temperatures into the atmosphere, forming clouds. Rain will fall. Raindrops will absorb CO2 and form carbonic acid. When the carbonic acid hits the ground, it will react with rock and dirt to exchange the carbon in the acid, for nitrogen in the rocks, causing rocks to become carbonates, and releasing gaseous nitrogen and oxygen. We can help this process along by releasing cyanobacteria into the lakes and seas of Mars, which feed on Co2 and release oxygen while forming their own carbonates that settle to the bottom of their seas to form limestone.
The fun part is that while pre-Montreal levels of chlorofluorocarbon production may seem a bit daunting, but the other chemical, carbon tetrafluoride, is over fifty times more powerful as a greenhouse gas than chlorofluorocarbon, so we can produce carbon tetrafluoride more easily at a lower rate of production to result in the same outcome, with the added benefit of no chlorine being released to operate as a free radical in the stratosphere, interfering in formation of ozone. Thus allowing ozone to form naturally, Mars will then have a natural defense — along with a thicker atmosphere — against a lot of solar radiation.
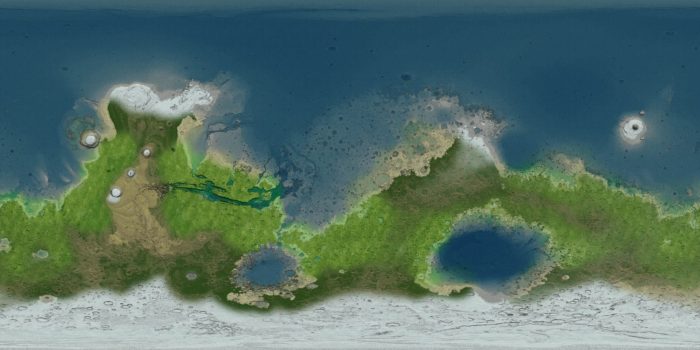
Also while chlorofluorocarbon can last for decades in the atmosphere, carbon tetrafluoride lasts for centuries, providing long term protection of the Martian climate greenhouse.
The final step is also dependent upon in situ resource utilization: building an electromagnetic field. Graphene is a superconductor. One can produce graphene by running CO2 through a magneto-hydro-dynamic furnace. By equipping robots with such abilities, constructing superconducting cable from atmospheric CO2, and setting the robots to traversing the Martian equator, reproducing themselves along the way, a superconducting coil can be assembled at the equator, powered by wind and solar power, to generate an electromagnetic shield for Mars.
While this is not a permanent solution, it can be maintained for centuries by robots. The ultimate solution will be to move one of the larger asteroids like Vesta or Psyche into Mars orbit, to provide a tidal influence upon Mars core that will restart its tectonic process that is the basis of Earth’s own magnetic field.
So, in conclusion, and back to the beginning, all of this begins with you, the dreamer, the explorer, the adventurer, who seeks new worlds, to build a new world, a new environment, a new economy and society, deciding that this is the future you want. Then to act to make it a reality, training to live and build in that reality. Come train at ISMuseum.org.
- Training to Inhabit Mars - July 31, 2021